|
- Greater genetic variability in Argentine Creole than in Thoroughbred horses based on serum protein polymorphisms
- S. Díaz, F.N. Dulout and P. Peral-García
- Centro de Investigaciones en Genética Básica y Aplicada (CIGEBA). Facultad de Ciencias Veterinarias, Universidad Nacional de La Plata (UNLP). 60 y 118 S/N. CC 296. B1900AVW
- La Plata, Argentina
- Corresponding author: P. Peral-García
- E-mail: [email protected]
- Genet. Mol. Res. 1 (3): 261-265 (2002)
- Received January 26, 2002
- Published September 23, 2002
ABSTRACT. Genetic polymorphism was analyzed for five blood proteins: albumin - Al, esterase - Es, a1B-glycoprotein - Xk, transferrin - Tf and hemoglobin - Hb in 200 Thoroughbred (TB) and 124 Argentine Creole (AC) horses. Of the five systems examined, Tf and Hb were not in Hardy-Weinberg equilibrium in either breed and Es was not in equilibrium in the Creole breed. Genetic variability, estimated as average heterozygosity, was higher in AC (H = 0.585 ± 0.131) than in TB (H = 0.353 ± 0.065). The genetic differentiation between these two populations (FST) was 0.109. Thus, of the total genetic differences between breeds, the proportion of genetic variation attributable to breed differences was about 10%; the remaining 90% was due to individual variation within breeds. The high degree of genetic variability seen in Argentine Creole horses could be a consequence of natural selection. Selection of TB through the centuries has most likely modified the gene pool of the ancestral population, with a consequent reduction in variability at certain loci. Probably, different mechanisms exist for maintaining polymorphism at these loci in TB and in AC horses. Heterozygosity may have played a fundamental role in adaptation.
Key words: Horses, Argentine Creole, Thoroughbred, Heterozygosity, Genetic variability, Serum protein polymorphysm
INTRODUCTION
Thoroughbred horses originated from a small group of North African and Middle Eastern horses were taken to England and bred for their racing ability. Arabian, Barb and Persian stallions were crossed with native mares, and made a definite contribution to Thoroughbred development. Genetic studies based on genealogical data have shown that, if all ancestors of contemporary horses with a contribution of ³1% are considered, only 21 individuals are responsible for 80% of the genes present in the modern Thoroughbred population (Cunningham, 1991).
The Argentine Creole horse breed is descended from the horses brought to the Americas by the Spanish conquerors. The descendants of these horses spread throughout America, and became wild horses called baguales or cimarrones in Argentine. At the end of the 19th century and the beginning of the 20th century, there was a renewed interest in recovering this breed, which had nearly disappeared through miscegenation with other breeds. This movement strived to restore the racial integrity of this breed by choosing sires with characteristic morphological features. The initial selective stage began with the establishment of the Selective and Genealogical Record, in 1939. Two important characteristics of the Creole breed are its hardiness, as shown by the ability to consume and assimilate any type of forage, and its resistance to and rapid recovery from fatigue. This breed also shows elevated fertility and longevity, and the animals are particularly strong (Dowdal, 1982). This breed also has phenotypic variability, but few studies (Peral García, 1994, 1996) have been done to determine the degree of genetic variability.
Biochemical polymorphisms are useful tools for evaluating genetic variability. The number and frequencies of alleles at different loci can be used to determine the genetic profile of a breed and to distinguish between individuals, populations and breeds. Genetic divergence among populations or breeds is usually measured by analysis of genetic distance and by fixation indexes (Wright, 1965). Nei (1977) and Wright (1978) concluded that all the fixation indices can be defined using the observed and expected heterozygosities at the equilibria of each population, when studying differentiation among populations of the same or different breeds.
We compared the genetic variability of Thoroughbred and Argentine Creole horses based on serum protein polymorphisms.
MATERIAL AND METHODS
Blood samples were obtained from 200 Thoroughbred and 124 Argentine Creole horses in the province of Buenos Aires. The individuals were chosen randomly from those registered by their respective official recording organization (Argentine Stud Book and Selective and Genealogical Record of Creole Horses). Electrophoresis techniques (Braend, 1967; Gahne et al., 1977; Juneja et al., 1978) were used to study the loci that codify the proteins: albumin - Al, esterase - Es, a1B-glycoprotein - Xk, transferrin - Tf and hemoglobin - Hb.
Measures of genetic variability such as the heterozygosity per locus (h) and average heterozygosity for joint loci (H) were determined by means of h = 1 - åxi2 and H = åh/r, respectively, where r is the number of loci and xi2 is the square of the frequency of each allele for the i loci. The variance of the average heterozygosity was estimated by V(H) = å(hj - H)2/(r - 1)/r, where å(hj - H)2/(r - 1) corresponds to V(h) or the heterozygosity per locus variance, as described by Crow and Kimura (1970) and Nei (1987).
The genetic differentiation between the horse breeds was analyzed by using fixation indices (Wright, 1965), modified by Nei (1977) and Wright (1978). The FIS and FIT statistics measure gene frequency differences by determining the excess or deficit of heterozygote phenotypes in each population and in the whole population, respectively. These statistics can have negative or positive values. The FST statistic measures the degree of differentiation among populations. Its value lies between zero and 1. The analyses were performed for each locus and for the mean of joint loci (Nei, 1987).
The deviations of FIS and FIT from zero were tested by the chi-square test c2 = NF2 (Hedrick, 1985). The significance of the genetic differences between populations (FST) was evaluated by a chi-square analysis [c2 = 2N FST (k - 1)] with (k - 1)(s - 1) degrees of freedom, where N is the total sample size, k is the number of alleles and s is the number of populations (Workman and Niswander, 1970; Chesser, 1983). The calculations were made using the statistical package BIOSYS (Version 1.7; Swofford and Selander, 1989).
RESULTS AND DISCUSSION
Of the five systems examined, Tf and Hb were not in Hardy-Weinberg equilibrium in either breed and Es was not in equilibrium in the Creole breed (Table 1). The observed fraction of heterozygotes for the five loci (H), was high in both breeds. In the Creole horses, the genetic variability was H = 0.585 (Table 2). Heterozygosity was greatest for the transferrin locus in both breeds. In Creole horses, about 70% of the individuals were heterozygotes for Tf and Es genotypes, with values of 0.4-0.6 at the other loci. In Thoroughbreds, the heterozygosity of the other systems was very diversified, with Xk showing a diversity of h = 0.01 (Table 2). The average heterozygosity is not representative of the actual situation at each locus, since important discontinuities were found among the diversity values of Thoroughbreds. The discontinuities indicate that the Thoroughbred population has a greater than expected variability.
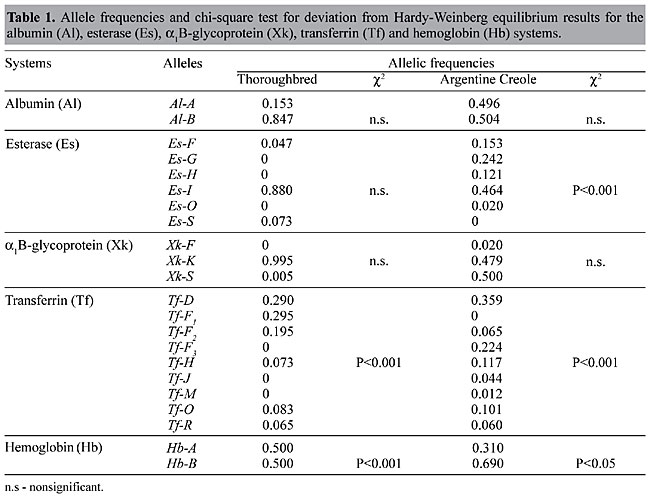
The prevalence of heterozygote phenotypes in Creole horses (Table 2) confirms the high degree of genetic diversity in this breed. Although there was extensive heterozygosity at each locus, the differences among the values of heterozygosity between loci were minimal. Although there is an important degree of genetic diversity among Creole horses, the average heterozygosity of a horse for those loci was similar for all individuals of this breed. Apparently there is some sort of mechanism for maintaining numerous allelic variants at these loci.
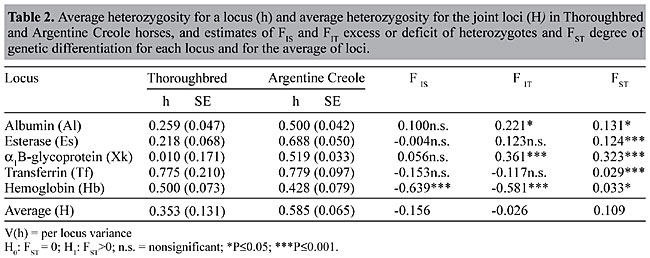
The FIT statistic (Table 2), calculated for the population as a whole (N = 324), indicated an overall increase of 2.6% in heterozygote phenotypes (FIT = -0.026) compared to homozygotes. The average excess of heterozygotes for each breed was 15.6% (FIS = -0.156). At each locus, in Tf, Es and Hb the fraction of heterozygotes exceeded the fraction of homozygotes, with significant values only for Hb (FIS = -0.639). For Al and Xk, the predominant phenotypes were homozygotes.
The degree of differentiation between breeds was highly significant for Xk (FST= 0.131), Es and Tf, and significant for Al and Hb (Table 2). The average genetic differentiation between breeds was 10.9% (FST = 0.109). This average estimate was similar to those determined for certain human races (FST = 0.088) (Nei and Roychoudhury, 1982), as well as breeds of Spanish dogs (FST = 0.099) (Jordana et al., 1992). The principal genetic differences at the population level (breed differences) were also about 10% (FST = 0.109) in our study. The remaining 90% of the total genetic variability was attributable to individual differences.
The heterozygosity of the hemoglobin loci in the two breeds and of the esterase and transferrin loci in Creole horses might have conferred some selective advantage on individuals bearing two different alleles. The genetic characteristics of these breeds reflect responses to environmental changes and to the type and magnitude of genetic variability originally present. The response to selection pressures apparently has favored maintenance of some multiallelic systems in Argentine Creole horses. Heterozygosity may have played a fundamental role in adaptation to new situations.
REFERENCES
Braend, M. (1967). Genetic variation of horse hemoglobin. Hereditas 58: 385-392.
Chesser, R.K. (1983). Genetic variability within and among populations of the black-tailed prairie dog. Evolution 37: 320-331.
Crow J.F. and Kimura, M. (1970). An Introduction to Population Genetics Theory. Alpha Editions, Burgess International Group Inc., New York, NY, USA.
Cunningham, P. (1991). The genetics of Thoroughbred horses. Sci. Am. 264: 92-98.
Dowdal, R.C. (1982). Criando Criollos. Editorial Hemisferio Sur S.A., Buenos Aires, Argentina.
Gahne, B., Juneja, R.K. and Grolmus, J. (1977). Horizontal polyacrylamide gel electrophoresis for the simultaneous phenotyping of transferrin, post-transferrin, albumin and post-albumin in the blood plasma of cattle. Anim. Blood Groups Biochem. Genet. 8: 127-137.
Hedrick, P.W. (1985). Genetics of Populations. Jones and Bartlet, Boston, MA, USA.
Jordana, J., Piedrafita, J., Sanchez, A. and Puig, P. (1992). Comparative F statistics of the genetic structure of ten Spanish dog breeds. J. Hered. 83: 367-374.
Juneja, R.K., Gahne, B. and Sandberg, K. (1978). Genetic polymorphism at the vitamin D binding protein and another post albumin protein in horse serum. Anim. Blood Groups Biochem. Genet. 9: 29-36.
Peral García, P. (1994). Caracterización racial de equinos de Pura Raza Criolla. Su comparación con los equinos de Pura Raza Española mediante el análisis de sus polimorfismos genéticos. Doctoral thesis, Facultad de Ciencias Naturales y Museo, Universidad Nacional de La Plata, La Plata, Argentina.
Peral García, P. (1996). Estudio de relaciones genéticas entre razas equinas mediante el análisis multivariado. Agrosur 24: 39-47.
Nei, M. (1977). F-statistics and analysis of gene diversity in subdivided populations. Ann. Hum. Genet. 41: 225-233.
Nei, M. (1987). Molecular Evolutionary Genetics. Columbia University Press, New York, NY, USA.
Nei, M. and Roychoudhury, A.K. (1982). Genetic relationship and evolution of human races. Evol. Biol. 14: 1-59.
Swofford, D.L. and Selander, R.B. (1989). A Computer Program for the Analysis of Allelic Variation in Population Genetics and Biochemical Systematics. D.L. Swofford, Illinois Natural History Survey, Champaign, IL, USA.
Workman P.L. and Niswander J.D. (1970). Population studies on southwestern Indian tribes. II. Local genetic differentiation in the Papago. J. Ann. Hum. Genet. 22: 24-49.
Wright, S. (1965). The interpretation of population structure by F-statistics with special regard to systems of mating. Evolution 19: 395-420.
Wright, S. (1978). Evolution and the Genetics of Populations: Vol. 4. Variability Within and Among Natural Populations. University of Chicago Press, Chicago, IL, USA.
|
|